Work has an eye on improving organ transplant
This story was first published on news.umbc.edu and was written by Sarah Hansen.
Embryos of zebrafish, a workhorse model organism in biology labs, routinely survive without oxygen for up to 50 hours by hitting the pause button on their development. Rachel Brewster, professor of biological sciences, and her team are working hard to learn how they do it. She hopes mechanisms that enable survival without oxygen could eventually be intentionally triggered in tissues like donated organs, to increase the length of time that can pass before they are transplanted.
That brief window of time is a major limiting factor in the availability of organs for transplant. Today, donated organs are cooled to slow metabolism and reduce oxygen consumption. Using that technique, hearts and lungs last only four to six hours, and kidneys up to 48. After that, tissues break down due to lack of cellular energy. This sliver of time to identify a recipient and deliver an organ means recipients are limited to a small geographic area in which to find a donor. Each day in the U.S., 79 people receive organ transplants and 22 die waiting for an organ.
Brewster’s research is supported by an Idea Discovery Grant from the U.S. Department of Defense (DoD). The DoD is interested in ways to improve transplant technology to increase the chances that injured soldiers would have timely access to donor organs and other tissues. If successful, the benefits would extend beyond the military.
The zebrafish Brewster work with “can arrest everything they’re doing as a mechanism to conserve cellular energy,” she says, so the goal is to “find the signaling mechanisms that shut down cellular activity.” Tissues survive by reducing energy consumption when energy production plummets in the absence of oxygen.
One of Brewster’s goals with the new project is to understand the timing of arrest—when development is paused—in zebrafish embryos. The research group has already learned that younger embryos arrest faster and more efficiently than older embryos. Also, older embryos are less likely to survive when oxygen is reintroduced.
Brewster would like to understand the sequence of arrest, as well. For example, does cell division in muscles arrest before it does in the digestive tract? Does tissue in an extremity like the tail succumb to a lack of oxygen before brain tissue? Are there essential systems that never arrest? “We can’t identify signaling mechanisms that cause arrest until we define arrest,” she says.
The team also hopes to identify small molecules whose concentrations in the cell change quickly upon oxygen deprivation, allowing them to mediate the rates of cellular processes on a short time scale. Brewster believes these molecules’ rapid effect on the cell makes them more likely to be involved in arrest than slower processes, such as the rate at which proteins are built from genetic instructions and then modified by cellular machinery.
Brewster hypothesizes that the involved small molecules activate or deactivate larger effector molecules, such as a class of proteins known as “kinases,” that carry out the arrest process. The team’s most ambitious goal is to identify those effector molecules. Rather than one “master regulator” molecule that controls the arrest process, Brewster explains, “It’s more likely every tissue fending for itself.” She adds, “My hunch is that the system is sloppier than we predicted.”
The experiments themselves are challenging and complex. The team will work with individual zebrafish embryos, and even using very young, fairly homogeneous embryos won’t make them all perfectly identical. Individual differences may show up as false positives in their results. “To figure out what is signal versus noise is not easy,” says Brewster.
One tack the team is taking to find molecules that trigger arrest is to knock out candidate molecules one by one. They’ll know they’ve got a winner if embryos without that molecule continue to develop in oxygen-deprived conditions.
“To actually identify a molecule that promotes arrest would be very exciting,” says Brewster. In addition to the organ transplant, another application of intentionally-triggered arrest might be during open heart surgery to prevent or slow tissue damage. Someday, NASA might use the technique to trigger a low-metabolic state in astronauts, similar to hibernation, to allow them to survive trips farther and farther from Earth.
Beyond practical applications, this project attracted Brewster on a more fundamental level. “Ninety-nine percent of developmental biologists study how development progresses,” she explains. “I was intrigued by the prospect of studying the exact opposite.”
Brewster is excited to share her passion for discovering how development can be paused with the many students who work in her lab, such as Ph.D. student Jong Park, who will focus on this project. Undergraduates have also contributed significantly to the research. “I’m very proud that most of the preliminary data was produced by undergrads,” she says. “It’s really kudos to them that we got this thing funded.”
Tags:
Posted: October 8, 2016, 1:39 PM
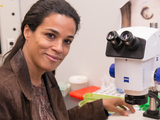